The language of covalent histone modifications
Histone proteins and the nucleosomes they form with DNA are the fundamental building blocks of eukaryotic chromatin. A diverse array of post-translational modifications that often occur on tail domains of these proteins has been well documented. Although the function of these highly conserved modifications has remained elusive, converging biochemical and genetic evidence suggests functions in several chromatin-based processes. We propose that distinct histone modifications, on one or more tails, act sequentially or in combination to form a ‘histone code’ that is, read by other proteins to bring about distinct downstream events.
This is a preview of subscription content, access via your institution
Access options
Subscribe to this journal
Receive 51 print issues and online access
196,21 € per year
only 3,85 € per issue
Buy this article
- Purchase on SpringerLink
- Instant access to full article PDF
Prices may be subject to local taxes which are calculated during checkout
Similar content being viewed by others
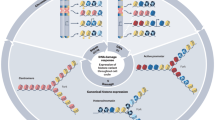
Multifunctional histone variants in genome function
Article 13 August 2024
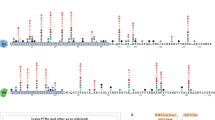
Histone post-translational modifications — cause and consequence of genome function
Article 25 March 2022
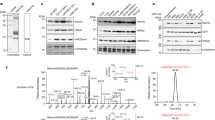
Acetyl-methyllysine marks chromatin at active transcription start sites
Article 20 September 2023
References
- Luger,K. & Richmond,T. J. The histone tails of the nucleosome. Curr. Opin. Genet. Dev.8, 140–146 (1998). ArticleCASPubMedGoogle Scholar
- Kornberg,R. D. & Lorch,Y. Twenty-five years of the nucleosome, fundamental particle of the eukaryotic chromosome. Cell98, 285–294 (1999). CASPubMedGoogle Scholar
- van Holde,K. E. in Chromatin (ed. Rich, A.) 111–148 (Springer, New York, 1988). Google Scholar
- Wolffe,A. P. & Hayes,J. J. Chromatin disruption and modification. Nucleic Acids Res.27, 711–720 (1999). ArticleCASPubMedPubMed CentralGoogle Scholar
- Hecht,A., Laroche,T., Strahl-Bolsinger,S., Gasser,S. M. & Grunstein,M. Histone H3 and H4 N-termini interact with SIR3 and SIR4 proteins: a molecular model for the formation of heterochromatin in yeast. Cell80, 583–592 (1995). ArticleCASPubMedGoogle Scholar
- Edmondson,D. G., Smith,M. M. & Roth,S. Y. Repression domain of the yeast global repressor Tup1 interacts directly with histones H3 and H4. Genes Dev.10, 1247–1259 (1996). ArticleCASPubMedGoogle Scholar
- Luger,K., Mader,A. W., Richmond,R. K., Sargent,D. F. & Richmond,T. J. Crystal structure of the nucleosome core particle at 2.8 Å resolution. Nature389, 251–260 (1997). ArticleADSCASPubMedGoogle Scholar
- Hansen,J. C., Tse,C. & Wolffe,A. P. Structure and function of the core histone N-termini: more than meets the eye. Biochemistry37, 17637–17641 (1998). ArticleCASPubMedGoogle Scholar
- Mizzen,C. et al. Signaling to chromatin through histone modifications: how clear is the signal? Cold Spring Harb. Symp. Quant. Biol.63, 469–481 (1998). ArticleCASPubMedGoogle Scholar
- Turner,B. M. Decoding the nucleosome. Cell75, 5–8 (1993). ArticleCASPubMedGoogle Scholar
- Lopez-Rodas,G. et al. Histone deacetylase. A key enzyme for the binding of regulatory proteins to chromatin. FEBS Lett.317, 175–180 (1993). ArticleCASPubMedGoogle Scholar
- Loidl,P. Histone acetylation: facts and questions. Chromosoma103, 441–449 (1994). ArticleCASPubMedGoogle Scholar
- Tordera,V., Sendra,R. & Perez-Ortin,J. E. The role of histones and their modifications in the informative content of chromatin. Experientia49, 780–788 (1993). ArticleCASPubMedGoogle Scholar
- Grunstein,M. Histone acetylation in chromatin structure and transcription. Nature389, 349–352 (1997). ArticleADSCASPubMedGoogle Scholar
- Struhl,K. Histone acetylation and transcriptional regulatory mechanisms. Genes Dev.12, 599–606 (1998). ArticleCASPubMedGoogle Scholar
- Thorne,A. W., Kmiciek,D., Mitchelson,K., Sautiere,P. & Crane-Robinson,C. Patterns of histone acetylation. Eur. J. Biochem.193, 701–713 (1990). ArticleCASPubMedGoogle Scholar
- Kuo,M. H. et al. Transcription-linked acetylation by Gcn5p of histones H3 and H4 at specific lysines. Nature383, 269–272 (1996). ArticleADSCASPubMedGoogle Scholar
- Grant,P. A. et al. Expanded lysine acetylation specificity of Gcn5 in native complexes. J. Biol. Chem.274, 5895–5900 (1999). ArticleCASPubMedGoogle Scholar
- Zhang,W., Bone,J. R., Edmondson,D. G., Turner,B. M. & Roth,S. Y. Essential and redundant functions of histone acetylation revealed by mutation of target lysines and loss of the Gcn5p acetyltransferase. EMBO J.17, 3155–3167 (1998). ArticleCASPubMedPubMed CentralGoogle Scholar
- Rojas,J. R. et al. Structure of Tetrahymena GCN5 bound to coenzyme A and a histone H3 peptide. Nature401, 93–98 (1999). ArticleADSCASPubMedGoogle Scholar
- Tanner,K. G. et al. Catalytic mechanism and function of invariant glutamic acid 173 from the histone acetyltransferase GCN5 transcriptional coactivator. J. Biol. Chem.274, 18157–18160 (1999). ArticleCASPubMedGoogle Scholar
- Trievel,R. C. et al. Crystal structure and mechanism of histone acetylation of the yeast GCN5 transcriptional coactivator. Proc. Natl Acad. Sci. USA96, 8931–8936 (1999). ArticleADSCASPubMedPubMed CentralGoogle Scholar
- Clements,A. et al. Crystal structure of the histone acetyltransferase domain of the human PCAF transcriptional regulator bound to coenzyme A. EMBO J.18, 3521–3532 (1999). ArticleCASPubMedPubMed CentralGoogle Scholar
- Lin,Y., Fletcher,C. M., Zhou,J., Allis,C. D. & Wagner,G. Solution structure of the catalytic domain of GCN5 histone acetyltransferase bound to coenzyme A. Nature400, 86–89 (1999). ArticleADSCASPubMedGoogle Scholar
- Sternglanz,R. & Schindelin,H. Structure and mechanism of action of the histone acetyltransferase gcn5 and similarity to other N-acetyltransferases. Proc. Natl Acad. Sci. USA96, 8807–8808 (1999). ArticleADSCASPubMedPubMed CentralGoogle Scholar
- Kimura,A. & Horikoshi,M. How do histone acetyltransferases select lysine residues in core histones? FEBS Lett.431, 131–133 (1998). ArticleCASPubMedGoogle Scholar
- Turner,B. M. & O'Neill,L. P. Histone acetylation in chromatin and chromosomes. Semin. Cell Biol.6, 229–236 (1995). ArticleCASPubMedGoogle Scholar
- Annunziato,A. T. in The Nucleus (ed. Wolffe, A. P.) 31–56 (JAI, Greenwich, Connecticut, 1995). BookGoogle Scholar
- Allis,C. D., Chicoine,L. G., Richman,R. & Schulman,I. G. Deposition-related histone acetylation in micronuclei of conjugating Tetrahymena. Proc. Natl Acad. Sci. USA82, 8048–8052 (1985). ArticleADSCASPubMedPubMed CentralGoogle Scholar
- Sobel,R. E., Cook,R. G., Perry,C. A., Annunziato,A. T. & Allis,C. D. Conservation of deposition-related acetylation sites in newly synthesized histones H3 and H4. Proc. Natl Acad. Sci. USA92, 1237–1241 (1995). ArticleADSCASPubMedPubMed CentralGoogle Scholar
- Tyler,J. K. et al. The RCAF complex mediates chromatin assembly during DNA replication and repair. Nature402, 555–560 (1999). ArticleADSCASPubMedGoogle Scholar
- Bradbury,E. M. Reversible histone modifications and the chromosome cell cycle. Bioessays14, 9–16 (1992). ArticleCASPubMedGoogle Scholar
- Koshland,D. & Strunnikov,A. Mitotic chromosome condensation. Annu. Rev. Cell Dev. Biol.12, 305–333 (1996). ArticleCASPubMedGoogle Scholar
- Mahadevan,L. C., Willis,A. C. & Barratt,M. J. Rapid histone H3 phosphorylation in response to growth factors, phorbol esters, okadaic acid, and protein synthesis inhibitors. Cell65, 775–783 (1991). ArticleCASPubMedGoogle Scholar
- Thomson,S., Mahadevan,L. C. & Clayton,A. L. MAP kinase-mediated signalling to nucleosomes and immediate-early gene induction. Semin. Cell Dev. Biol.10, 205–214 (1999). ArticleCASPubMedGoogle Scholar
- Chadee,D. N. et al. Increased Ser-10 phosphorylation of histone H3 in mitogen-stimulated and oncogene-transformed mouse fibroblasts. J. Biol. Chem.274, 24914–24920 (1999). ArticleCASPubMedGoogle Scholar
- Sassone-Corsi,P. et al. Requirement of Rsk-2 for epidermal growth factor-activated phosphorylation of histone H3. Science285, 886–891 (1999). ArticleCASPubMedGoogle Scholar
- De Cesare,D., Jacquot,S., Hanauer,A. & Sassone-Corsi,P. Rsk-2 activity is necessary for epidermal growth factor-induced phosphorylation of CREB protein and transcription of c-fos gene. Proc. Natl Acad. Sci. USA95, 12202–12207 (1998). ArticleADSCASPubMedPubMed CentralGoogle Scholar
- Thomson,S. et al. The nucleosomal response associated with immediate-early gene induction is mediated via alternative MAP kinase cascades: MSK1 as a potential histone H3/HMG-14 kinase. EMBO J.18, 4779–4793 (1999). ArticleCASPubMedPubMed CentralGoogle Scholar
- Jin,Y. et al. JIL-1: a novel chromosomal tandem kinase implicated in transcriptional regulation in Drosophila. Mol. Cell4, 129–135 (1999). ArticleCASPubMedGoogle Scholar
- Lucchesi,J. C. Dosage compensation in flies and worms: the ups and downs of X-chromosome regulation. Curr. Opin. Genet. Dev.8, 179–184 (1998). ArticleCASPubMedGoogle Scholar
- Turner,B. M., Birley,A. J. & Lavender,J. Histone H4 isoforms acetylated at specific lysine residues define individual chromosomes and chromatin domains in Drosophila polytene nuclei. Cell69, 375–384 (1992). ArticleCASPubMedGoogle Scholar
- von Holt,C. et al. Isolation and characterization of histones. Methods Enzymol.170, 431–523 (1989). ArticleCASPubMedGoogle Scholar
- Strahl,B. D., Ohba,R., Cook,R. G. & Allis,C. D. Methylation of histone H3 at lysine 4 is highly conserved and correlates with transcriptionally active nuclei in Tetrahymena. Proc. Natl Acad. Sci. USA96, 14967–14972 (1999). ArticleADSCASPubMedPubMed CentralGoogle Scholar
- Chen,D. et al. Regulation of transcription by a protein methyltransferase. Science284, 2174–2177 (1999). ArticleCASPubMedGoogle Scholar
- Nakajima,T. et al. The signal-dependent coactivator CBP is a nuclear target for pp90RSK. Cell86, 465–474 (1996). ArticleCASPubMedGoogle Scholar
- Berger,S. L. Gene activation by histone and factor acetyltransferases. Curr. Opin. Cell Biol.11, 336–341 (1999). ArticleCASPubMedGoogle Scholar
- Cosma,M. P., Tanaka,T. & Nasmyth,K. Ordered recruitment of transcription and chromatin remodeling factors to a cell cycle- and developmentally regulated promoter. Cell97, 299–311 (1999). ArticleCASPubMedGoogle Scholar
- Krebs,J. E., Kuo,M. H., Allis,C. D. & Peterson,C. L. Cell cycle-regulated histone acetylation required for expression of the yeast HO gene. Genes Dev.13, 1412–1421 (1999). ArticleCASPubMedPubMed CentralGoogle Scholar
- Clark,D. et al. Chromatin structure of transcriptionally active genes. Cold Spring Harb. Symp. Quant. Biol.58, 1–6 (1993). ArticleCASPubMedGoogle Scholar
- Roth,S. Y. & Allis,C. D. Chromatin condensation: does histone H1 dephosphorylation play a role? Trends Biochem. Sci.17, 93–98 (1992). ArticleCASPubMedGoogle Scholar
- Barratt,M. J., Hazzalin,C. A., Cano,E. & Mahadevan,L. C. Mitogen-stimulated phosphorylation of histone H3 is targeted to a small hyperacetylation-sensitive fraction. Proc. Natl Acad. Sci. USA91, 4781–4785 (1994). ArticleADSCASPubMedPubMed CentralGoogle Scholar
- Hendzel,M. J. Mitosis-specific phosphorylation of histone H3 initiates primarily within pericentromeric heterochromatin during G2 and spreads in an ordered fashion coincident with mitotic chromosome condensation. Chromosoma106, 348–360 (1997). ArticleCASPubMedGoogle Scholar
- Wei,Y., Yu,L., Bowen,J., Gorovsky,M. A. & Allis,C. D. Phosphorylation of histone H3 is required for proper chromosome condensation and segregation. Cell97, 99–109 (1999). ArticleCASPubMedGoogle Scholar
- Goto,H. et al. Identification of a novel phosphorylation site on histone H3 coupled with mitotic chromosome condensation. J. Biol. Chem.274, 25543–25549 (1999). ArticleCASPubMedGoogle Scholar
- Sullivan,K. F., Hechenberger,M. & Masri,K. Human CENP-A contains a histone H3 related histone fold domain that is required for targeting to the centromere. J. Cell Biol.127, 581–592 (1994). ArticleCASPubMedGoogle Scholar
- Hirano,T. SMC-mediated chromosome mechanics: a conserved scheme from bacteria to vertebrates? Genes Dev.13, 11–19 (1999). ArticleCASPubMedGoogle Scholar
- De Rubertis,F. et al. The histone deacetylase RPD3 counteracts genomic silencing in Drosophila and yeast. Nature384, 589–591 (1996). ArticleADSCASPubMedGoogle Scholar
- Braunstein,M., Sobel,R. E., Allis,C. D., Turner,B. M. & Broach,J. R. Efficient transcriptional silencing in Saccharomyces cerevisiae requires a heterochromatin histone acetylation pattern. Mol. Cell. Biol.16, 4349–4356 (1996). ArticleCASPubMedPubMed CentralGoogle Scholar
- Dhalluin,C. et al. Structure and ligand of a histone acetyltransferase bromodomain. Nature399, 491–496 (1999). ArticleADSCASPubMedGoogle Scholar
- Winston,F. & Allis,C. D. The bromodomain: a chromatin-targeting module? Nature Struct. Biol.6, 601–604 (1999). ArticleCASPubMedGoogle Scholar
- Pawson,T. Protein modules and signalling networks. Nature373, 573–580 (1995). ArticleADSCASPubMedGoogle Scholar
- Roberts,S. M. & Winston,F. Essential functional interactions of SAGA, a Saccharomyces cerevisiae complex of Spt, Ada, and Gcn5 proteins, with the Snf/Swi and Srb/mediator complexes. Genetics147, 451–465 (1997). CASPubMedPubMed CentralGoogle Scholar
- Biggar,S. R. & Crabtree,G. R. Continuous and widespread roles for the Swi–Snf complex in transcription. EMBO J.18, 2254–2264 (1999). ArticleCASPubMedPubMed CentralGoogle Scholar
- Sudarsanam,P., Cao,Y., Wu,L., Laurent,B. C. & Winston,F. The nucleosome remodeling complex, Snf/Swi, is required for the maintenance of transcription in vivo and is partially redundant with the histone acetyltransferase, Gcn5. EMBO J.18, 3101–3106 (1999). ArticleCASPubMedPubMed CentralGoogle Scholar
- Georgel,P. T., Tsukiyama,T. & Wu,C. Role of histone tails in nucleosome remodeling by Drosophila NURF. EMBO J.16, 4717–4726 (1997). ArticleCASPubMedPubMed CentralGoogle Scholar
- Luduena,R. F. Multiple forms of tubulin: different gene products and covalent modifications. Int. Rev. Cytol.178, 207–275 (1998). ArticleCASPubMedGoogle Scholar
- Luduena,R. F., Banerjee,A. & Khan,I. A. Tubulin structure and biochemistry. Curr. Opin. Cell Biol.4, 53–57 (1992). ArticleCASPubMedGoogle Scholar
- Nogales,E., Whittaker,M., Milligan,R. A. & Downing,K. H. High-resolution model of the microtubule. Cell96, 79–88 (1999). ArticleCASPubMedGoogle Scholar
Acknowledgements
Research surrounding this topic in our laboratory is supported by grants from the NIH to C.D.A. and B.D.S. We wish to thank current laboratory members, especially C. A. Mizzen, for critical review of this manuscript, and T. K. Archer and C. L. Smith for kindly sharing unpublished data.
Author information
Authors and Affiliations
- Department of Biochemistry and Molecular Genetics, University of Virginia Health Science Center, Charlottesville, 22908, Virginia, USA Brian D. Strahl & C. David Allis
- Brian D. Strahl